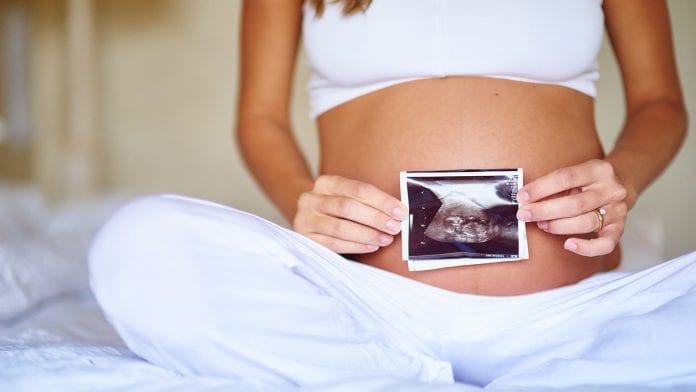
Researchers grew a three-dimensional organoid model of the human placenta – an achievement carrying significant implications for placental disorders.
Defective placentation is the underlying cause of various pregnancy complications. Studies on human placental development and disorders are hampered by the lack of a human in vitro model that would recapitulate formation of trophoblast progenitors and differentiated subtypes, in a correct 3D orientation. Now, a team from the Medical University of Vienna have taken on the challenge and derived a model of the trophoblast portion of the human placenta that mimics for the first time aspects of trophoblast tissue structure in a dish, with important implications for placental disorders.1
Understanding the placenta
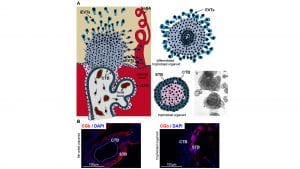
The placenta is an organ that provides an interface between the maternal and the foetal bloodstreams and serves as the site of exchange of nutrients, gases, metabolites, hormones, and other substances that are essential for embryonic development.2 The function of the placenta is to bring the two bloodstreams into close proximity and facilitate the exchange between them through a variety of highly specialised trophoblast cell types.
These trophoblast subtypes are derived from a common progenitor population called cytotrophoblast (CTB). CTB gives rise to two major differentiated trophoblast cell types: syncytiotrophoblast (STB) and extravillous trophoblast (EVT) (Fig. 1a). STB results from the fusion of CTB cells into the multinucleated structure. STB is in direct contact with the maternal blood and is thus intimately involved in maternal/foetal exchange but also in the maintenance of pregnancy as it produces key hormones such as human chorionic gonadotrophin, measured in pregnancy tests (Fig. 1b).
The second subtype, EVTs, is highly invasive and can be further subdivided into those that invade the uterine endometrium (interstitial iEVTs) and those that invade and remodel maternal spiral arteries (endovascular eEVTs) (Fig.1a).3 The molecular mechanisms that orchestrate the co-ordinated CTB progenitor differentiation and specialisation into STB and EVTs are poorly understood, not least due to the lack of a reliable human experimental system.
The challenge: placental disorders
According to the United Nations Population Fund, 830 women die every day (about one woman every two minutes) from causes related to pregnancy or childbirth (www.unfpa.org). Major pregnancy complications including miscarriage, pre-eclampsia, intrauterine growth restriction (IUGR) and stillbirth have been attributed to poor placentation. In particular, shallow trophoblast invasion and failure in the modification of the maternal vasculature are pathological features of pre-eclampsia and other pregnancy disorders including early pregnancy loss, IUGR, pre-term birth and placenta abruption.4
To develop successful therapies, we need to first decipher the molecular mechanisms co-ordinating invasive trophoblast differentiation and remodelling of maternal uterine arteries.
However, our molecular understanding of the aetiology of placental disorders is limited, not least due to a lack of robust human model systems. While model organisms (e.g. mouse) only partially recapitulate human placental development and function, human placental explants in vitro, primary culture and transformed trophoblast cell lines have severe limitations.5 In addition, the restricted availability of human tissue and ethical concerns hamper progress in studying human placental development and disease.
The solution: organoids
Recent years have witnessed an unprecedented advance in biomedical research with the advent of self-organising 3D tissue in vitro models, called organoids.6,7 They have been derived from a wide variety of organs, including the intestine, stomach, liver, lung, brain, retina, kidney and uterus. Organoid culture relies on self-organisation and is established by embedding progenitor cells into a jelly substance that mimics the extracellular matrix and provides an external scaffold. The self-organisation results in tissue-specific spatial organisation of cell types as well as a dynamic balance between self-renewing progenitor cells and differentiated specialised cells.
Organoids comprise only a few cell types (usually epithelial) of an actual organ and are therefore simpler to study and analyse. As a consequence, we are in the midst of an ongoing revolution in biomedical science that is bringing us closer to deciphering mechanisms of human development and disorders, the design of new drugs, and tailored regenerative medicine.6,7
For instance, cancer patient-derived organoids are being used to screen for optimised and personalised drug combinations to treat colorectal and breast cancer as well as genetic diseases such as cystic fibrosis. Moreover, these patient-derived organoids are deposited in large biobanks and used for development of new drugs and toxicity tests.
The Medical University of Vienna and placental disorders
Given all the advantages of organoid technology and the lack of human trophoblast models, a team from the Medical University of Vienna took on the challenge to close this gap and provide the community with a patient-derived, physiological, self-renewing and genetically modifiable placental model.
The team of researchers was led by Professor Martin Knöfler, a world expert in human trophoblast biology, and Dr Sandra Haider from the Department of Obstetrics and Gynecology, as well as Dr Paulina Latos, a stem cell biologist from the Center for Anatomy and Cell Biology.
Recalling the beginning of this collaboration, Latos says: “During my postdoctoral stay at the University of Cambridge (UK), I was exposed to a number of great labs using this new exciting organoid technology. I was very fortunate and excited that, after moving to Vienna, an eminent expert in human placental research, Professor Martin Knöfler, agreed to collaborate, team up and pool together our expertise to derive human trophoblast organoids.
Towards a three-dimensional organoid model
The team from Vienna first isolated the progenitor population from sixth- to seventh-week placentae and embedded them in an extracellular matrix. Based on other organoid media, the researchers optimised the cocktail of growth factors and signalling inhibitors that promoted expansion and long-term growth of trophoblast organoids.
Global gene expression profiling and comparison to the placental tissue of origin revealed that the organoids express markers of both the progenitor population (CTB) as well as differentiated STB.1 The analysis of the 3D structures revealed an outer proliferative CTB layer. Towards the centre of the organoids, the CTB cells differentiated and spontaneously underwent cell fusion, giving rise to functionally active, hormone-secreting STB, mimicking placental development (Fig. 1b). Defined changes in the media composition decreased CTB self-renewal and induced development of proliferative, invasive and migratory EVT.1
Taken together, these in vitro structures developed in a 3D arrangement contained all three major trophoblast cell types and mimicked the in vivo steps of placenta formation and differentiation.
Future implications
Newly developed medical treatments for human disease usually have limitations such as individual differences among patients, difficulties with prediction of outcomes, and time-consuming drug testing. Importantly, treatment of placental disorders is particularly challenging given that drugs cannot be tested in pregnant women.
Trophoblast organoid cultures based on a specific disease and on a specific individual are expected to develop into powerful tools for precision therapy. They may become useful for studying placental transport, drug testing, gene editing, or research on prognosis. Future screens may be performed using trophoblast organoid biobanks with the aim to identify both new drugs and patients who may benefit from treatment with existing drugs. Furthermore, trophoblast organoids may be used in the future for toxicology testing to complement, or even in part replace, animal testing.
Overall, given that trophoblast organoids have the capacity for rapid growth and stable differentiation and are suitable for genetic manipulations using CRISPR/Cas9, they have the potential to become a powerful tool for modelling placental disorders, toxicology tests and development of precision therapy.
Knöfler summarises: “As the causes of human placental disorders were notoriously difficult to study, the derivation of placental organoids will pave the way for future drug development and medical treatments.”
References
- Haider S, Meinhardt G, Saleh L, Kunihs V, Gamperl M, Kaindl U, Ellinger A, Burkard TR, Fiala C, Pollheimer J, Mendjan S, Latos PA, Knöfler M. Self-Renewing Trophoblast Organoids Recapitulate the Developmental Program of the Early Human Placenta. Stem Cell Reports. 2018;11(2):537-51
- Burton GJ, Fowden AL. The placenta: a multifaceted, transient organ. Philos Trans R Soc Lond B Biol Sci. 2015;370(1663):20140066
- Bischof P, Irminger-Finger I. The human cytotrophoblastic cell, a mononuclear chameleon. Int J Biochem Cell Biol. 2005;37(1):1-16
- Kaufmann P, Black S, Huppertz B. Endovascular trophoblast invasion: implications for the pathogenesis of intrauterine growth retardation and preeclampsia. Biol Reprod. 2003;69(1):1-7
- Soares MJ, Varberg KM, Iqbal K. Hemochorial placentation: development, function, and adaptations. Biol Reprod. 2018;99(1):196-211
- Artegiani B, Clevers H. Use and application of 3D-organoid technology. Hum Mol Genet. 2018;27(R2):R99-R107
- Drost J, Clevers H. Translational applications of adult stem cell-derived organoids. Development. 2017;144(6):968-75
Professor Martin Knöfler and Dr Sandra Haider
Department of Obstetrics
and Gynaecology
Reproductive Biology Unit
Dr Paulina Latos
Center for Anatomy and Cell Biology
Medical University of Vienna
+43 1 40400 28420
+43 1 40160 37718
martin.knoefler@meduniwien.ac.at
sandra.haider@meduniwien.ac.at
paulina.latos@meduniwien.ac.at
This article will appear in issue 7 of Health Europa Quarterly, which will be published in November 2018.