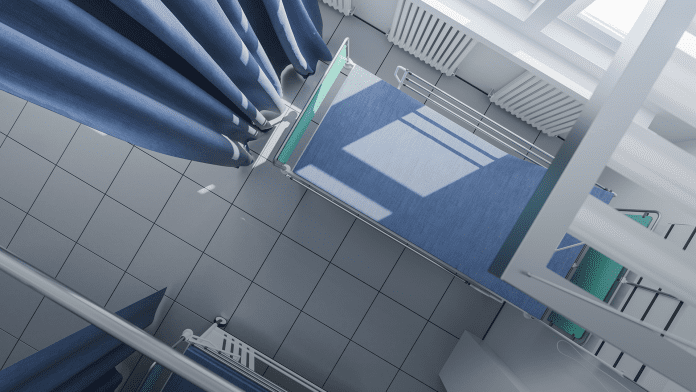
An overview of environmental hygiene trends, challenges, interventions and best practices as found in recent medical literature.
As the COVID-19 pandemic rages on, the role of environmental hygiene in preventing and slowing the spread of infection remains a singularly pressing concern within healthcare facilities. This review collates and investigates key recent findings within the medical field pertaining to environmental hygiene challenges, interventions and best practice.
The importance of cleaning
Moore et al. (2020) conducted environmental sampling in eight English hospitals during the ‘first wave’ of the pandemic, in order to assess the presence of aerosolised viral particles in the air and surface contamination in areas near symptomatic and asymptomatic patients. After analysing samples using viral isolation assays and reverse transcription polymerase chain reaction (RT-PCR) tests, the team reported that 30 of the 336 surfaces studied had COVID-19 RNA present – although overall bacterial counts were low, indicating that the hospitals’ cleaning protocols were largely effective. Of the 55 air samples taken from spaces close to patients, four contained COVID-19 RNA in low concentrations. None of the samples which tested positive for the virus were shown to be infectious.
The study’s authors stated: “Effective cleaning can reduce the risk of fomite (contact) transmission but some surface types may facilitate the survival, persistence and/or dispersal of SARS-CoV-2. The presence of low or undetectable concentrations of viral RNA in the air supports current guidance on the use of specific PPE ensembles for aerosol and non-aerosol generating procedures.”
Routes of transmission
Redmond et al. (2020) highlight the need for wider understanding of the paths and means by which pathogens are transmitted, in order to develop effective hygiene protocols; particularly with regard to healthcare-associated and antimicrobial-resistant pathogens. To best identify how and by what routes bacteria were transferred within clinical spaces, the researchers tracked bacterial contamination in the rooms of 17 patients newly admitted to the hospital. Each room was fully cleaned and sanitised prior to the study; and all the patients tested negative for methicillin-resistant Staphylococcus aureus (MRSA) and other HAIs.
The researchers monitored patients’ movements, recording how they interacted with staff and equipment. They collected daily bacterial cultures from high-touch surfaces, beds, patients’ socks and various sections of each room’s floor, as well as from the patients themselves. Within 24 hours of the patients’ admission, just under half of the rooms had tested positive for the presence of MRSA; within four days, 58% of the rooms showed positive tests for MRSA, Clostridium difficile (C. difficile) or vancomycin-resistant enterococci (VRE). The data appeared to indicate that pathogen contamination had typically begun on the floors of patients’ rooms from which pathogens were transferred to patients’ socks, bedding and surfaces.
Floor contamination
Observation of the timing and location of the emergence of MRSA contamination on floors indicated that pathogens were carried into the rooms on the footwear of hospital staff. The authors of the study highlighted the rapid accumulation of HAI pathogens on the floors of patients’ rooms and the attendant risk of transferal around the room, to patients’ socks, bedding and high-touch surfaces, noting that the floors of healthcare facilities may be ‘underappreciated’ as vectors of infection.
A study published in the August 2020 edition of the Infection Control & Hospital Epidemiology journal showed similar results with regards to COVID-19 contamination, with traces of the virus frequently detected on the floor of a COVID-19 ward, as well as on the shoes of staff who had entered the ward. The study’s authors identified a need for further targeted research into the role of floor contamination in bacterial and viral transmission in order to determine the best ways to combat the spread of infection through this route.
Mobile device transmission
Simmonds (2020) cautioned: “The undisputed versatility of smart devices makes them integral to modern-day society, especially within our health system. Despite the benefits, there are increasing concerns regarding their contamination and the associated infection risk. Bacteria under antimicrobial selective pressure can rapidly acquire resistant mechanisms leading to the assumption; mobile phones used within clinical environments may harbour bacteria associated with a higher infection mortality rate.”
Simmonds and her team deployed next-generation ‘metabarcoding’ sequencing technology to assess the levels of bacterial contamination on hospital staff members’ mobile devices: over a period of six months, the phones of 250 hospital personnel and 191 control subjects were swabbed for pathogens. While nearly all the devices studied were contaminated to some degree irrespective of their environment, of the 198 bacterial genera detected across the hospital and control groups, 34 were unique to the hospital employees’ phones. The hospital phones also displayed significantly higher levels of bacillus, MRSA and VRE contamination. The team drew attention to the clinical benefits of pathogen detection using metabarcoding rather than culture-based testing, noting that the sequencing method revealed a greater diversity of bacteria and that, by comparison, culture swabbing may not provide a wholly accurate picture of pathogenic contamination of devices.
Simmonds said: “Metabarcoding reinforces the need for mobile phone infection control practices to mitigate the risks associated with the increased use of smart device technology in clinical environments. These devices are currently exposing immunocompromised patients to unknown levels of pathogenic and multidrug-resistant bacteria. Departmental differences may suggest that the mobile phone is not just an extension to its owners but to their environment and that routine decontamination should be required to prevent the undermining of hand hygiene and the transmission of pathogenic bacteria.”
Sharing may not be caring
When a local medical facility announced that it would make a full-scale transition from offering primarily multiple-occupancy patient rooms to providing solely single-patient ensuite rooms, van der Schoor et al. (2020) identified a ‘unique’ opportunity to assess differing levels of environmental contamination between single- and multiple-patient spaces. The team conducted environmental sampling of 13 high-touch locations in 40 patient rooms around the hospital twice before the renovation and 12 times in the new building, beginning two weeks before the facility was due to reopen and conducting the final sample 15 months after patients were moved into the new space.
Patients and patient rooms were tested for methicillin-susceptible Staphylococcus aureus (MSSA), MRSA and highly resistant microorganisms (HRMOs) including Pseudomonas aeruginosa, Acinetobacter baumannii, and Enterococcus faecium. Of the multiple-patient rooms in the old building, 29 of 724 locations sampled (4%) indicated HRMO contamination; meanwhile, of the 3,358 locations sampled in the new building, only four tested positive (0.1%).
The team concluded: “The transition from mainly two- and four-person rooms to 100% single-patient rooms resulted in a significant decrease in environmental contamination…future sampling is needed to determine whether the low environmental contamination is a long-term effect of the transition to single rooms.”
A focus on C. difficile
Carling, et al. (2020) investigated the potential of a model environmental services (EVS) programme, deployed across eight hospitals, to combat the endemic spread of hospital-onset C. difficile infection (HO-CDI). In 2017, each hospital to be observed in the study independently implemented identical environmental hygiene interventions, comprising the targeted deployment of sporicidal surface disinfectant alongside a structured model EVS cleaning protocol. The EVS programme included key nodes such as optimised disinfection techniques, an updated training procedure for staff training, and an auditing system featuring objective performance feedback.
The study compared each hospital’s standardised infection ratio (SIR) from 18 months before the implementation of the programme with the SIRs to 12 months after implementation and reported individual site improvements between 20% and 92%. The team commented: “Overall, HO-CDI SIRs decreased almost 60% in the study hospitals following daily sporicidal disinfection cleaning of all patient-zone surfaces in association with ongoing programmatic optimisation of cleaning practice. As predicted by earlier single-site studies reporting a favourable impact of sporicidal disinfectant cleaning in outbreak settings, this multi-site quasi-experimental study has illustrated the substantial potential impact of hospital-wide sporicidal disinfection integrated with objectively sustained optimised thoroughness of cleaning to decrease the incidence of HO-CDI.”
Assessing ICUs
While it might seem reasonable to assume that intensive care units (ICUs) are particular vectors of infection, the available data would appear to suggest that this may not be the case. A study into environmental contamination of ICUs by Ong et al. (2020) assessed levels of ICU contamination and their correlation with disease factors, patient statuses and external considerations such as ventilation modalities. The study, which entailed the collection and testing of samples from surfaces in ICU patient rooms and common spaces, revealed that the level of environmental contamination within the ICU was overall lower than that of general patient wards.
The researchers noted: “The use of mechanical ventilation or high-flow nasal oxygen was not associated with greater surface contamination, supporting their use and safety from an infection control perspective. Transmission risk via environmental surfaces in the ICUs is likely to be low. Nonetheless, infection control practices should be strictly reinforced, and transmission risk via droplet or airborne spread remains.”
Environmental surveillance
The drug-resistant yeast Candida auris (C. auris) has been increasingly prevalent in the New York City metropolitan area since early 2019, with around half of all the USA’s C. auris diagnoses located in New York City. Solomon et al. (2020) set out to develop a comprehensive environmental surveillance programme to detect the presence of C. auris contamination within hospital spaces and assess the efficacy of various forms of post-discharge patient room cleaning methods.
The researchers sampled high-touch surfaces in patient rooms, including bedframes and over-bed tables; call buttons; privacy curtains; and oxygen meters, over a year-long period. They found that the terminal cleaning of the surfaces included in the study had an overall fail rate of 6.5%, with a significant discrepancy between cleaning methods: the traditional cleaning process, applying peracetic acid, hydrogen peroxide or bleach with microfibre cloths, led to an average fail rate of 9%; while a newer method of applying sodium dichloroisocyanurate (NaDCC) with microfibre cloths and electrostatic sprayers resulted in an average fail rate of only 2.5%. The study’s authors commented: “A systematic environmental surveillance system is critical for healthcare systems to assess touch-free disinfection and identify MDRO contamination of surfaces.”
Assessing interventions
In their 2020 study, Doll et al. explored the varying efficacies of five key infection control interventions aimed at decreasing rates of C. difficile in hospitals between 2013 and 2019. The team assessed the following interventions:
- ‘Two-step’ cleaning, whereby each iteration of daily and terminal cleaning in rooms of patients with C. difficile was performed twice: once with a quaternary ammonium product, followed by a second round of cleaning using a 10%
- bleach dilution;
- The deployment of UVC radiation for terminal room cleaning of all C. difficile patients;
- Implementing ‘contact plus’ patient
- isolation measures;
- Cleaning all patient areas using sporicidal peroxyacetic acid and hydrogen peroxide; and
- Adopting a decision support tool supported by electronic medical records (EMRs), to facilitate the appropriate ordering of C. difficile testing.
The results of the study showed little to no impact on rates of HO-CDI from the environmental cleaning interventions or ‘contact plus’ isolation protocols. The EMR-based decision support tool, however, was found to decrease the hospital’s HO-CDI rate by 5.1 per 10,000 patient days when adjusted for test volume. The researchers described the minimal impact of the various interventions as ‘disappointing’, adding: “This study adds to existing data that outside of an outbreak situation, traditional infection control guidance for CDI prevention has little impact on endemic rates.”
The importance of detailed cleaning
When the healthcare facility where they worked experienced a two-year outbreak of carbapenemase-producing Serratia marcescens (CPSM), Kwong et al. (2020) investigated potential sources and found a reservoir of the bacilli in the sink drains of clinical hand basins (CHB). The role of the sink drain population in furthering transmission was confirmed using whole-genome sequencing (WGS); and once CHBs had been positively identified as a key source of CPSM, the hospital was able to institute a facility-wide annual CHB cleaning intervention, involving manual scrubbing of the sink drains and connected pipes. Pre- and post-intervention point prevalence surveys (PPS) of CHB drains were implemented to assess further contamination.
The researchers concluded that: “A simple intervention targeted at reducing the biological burden of CPSM in CHB drains at regular intervals was effective in preventing transmission of carbapenemase-producing Enterobacterales from the hospital environment to patients over a prolonged period of intensive surveillance. These findings highlight the importance of detailed cleaning for controlling the spread of multidrug-resistant organisms from healthcare environments.”
References
- Pryek, KM. Environmental hygiene: trends from the medical literature. Healthcare Hygiene Magazine Vol. 3, Issue 1. January 2021. Pp. 12-20.
- Carling P, O’Hara L, et al. Mitigating Hospital-Onset C. difficile: Evaluation of a Standardised Environmental Hygiene Programme in Eight Hospitals. Infect Control Hosp Epidemiol. Vol. 41, Issue S1: The Sixth Decennial International Conference on Healthcare-Associated Infections Abstracts, March 2020: Global Solutions to Antibiotic Resistance in Healthcare. October 2020. Pp. s43. DOI: https://doi.org/10.1017/ice.2020.525
- Doll ME, Zhao J, et al. Chasing the rate: An interrupted time series analysis of interventions targeting reported hospital onset C. difficile, 2013-2018. Infect Control Hosp Epidemiol. Vol. 41, Issue 10. October 2020. Pp. 1142-1147. DOI: https://doi. org/10.1017/ice.2020.247
- Fakih MG, et al. Overcoming COVID-19: Addressing the perception of risk and transitioning protective behaviors to habits. Infect Control Hosp Epidemiol. Pp. 1-2. June 9, 2020. DOI: https:// doi.org/10.1017/ice.2020.284
- Kwong J, Leroi M, et al. A Simple Cleaning Intervention to Prevent Transmission of Carbapenemase-Producing Enterobacterales from Hospital Sinks. Infect Control Hosp Epidemiol. Vol. 41, Issue S1. October 2020. Pp. s103-s104.
- Moore G, Rickard H, et al. Detection of SARS-CoV-2 within the healthcare environment: a multicentre study conducted during the first wave of the COVID-19 outbreak in England. J Hosp Infect. November 28, 2020. DOI: https://doi.org/10.1016/j.jhin.2020.11.024
- Ong SWX, et al. Environmental contamination in a coronavirus disease 2019 (COVID-19) intensive care unit—What is the risk? Infect Control Hosp Epidemiol. Pp. 1-9. DOI: https://doi.org/10.1017/ ice.2020.1278
- Redmond S, Cadnum J, et al. Timing and Route of Contamination of Patient Rooms With Healthcare-Associated Pathogens. Infect Control Hosp Epidemiol. Vol. 41, Issue S1. October 2020, pp. s412. DOI: https:// doi.org/10.1017/ice.2020.1066
- Simmonds R. The Microbiome Analysis of Hospital Mobile Phones: Hidden Contaminants Revealed. Infect Control Hosp Epidemiol. Volume 41, Issue S1. October 2020. Pp. s409. DOI: https://doi.org/10.1017/ ice.2020.1061
- Solomon S, Phillips M, et al. The Development of an Environmental Surveillance Protocol to Detect Candida auris and Measure the Adequacy of Discharge Room Cleaning Performed by Different Methods. Infect Control Hosp Epidemiol. Vol. 41, Issue S1. October 2020. Pp. s404-s405. DOI: https:// doi.org/10.1017/ice.2020.1054
- van der Schoor AS, et al. 100% Single-Patient Rooms and Environmental Contamination With Highly Resistant Microorganisms: The MOVE Study. Infect Control Hosp Epidemiol. Vol. 41, Issue S1. October 2020. p. s91-s92. DOI: https:// doi.org/10.1017/ice.2020.588
Nova Biologicals
Dr Paul Pearce
nova@novatx.com
www.novatx.com
This article is from issue 16 of Health Europa. Click here to get your free subscription today